Beam-Plasma Interaction
Plasmas - in which matter is ionized, or "broken down" - are a natural environment in which to envision the future ultra-high gradient particle accelerator, as plasma waves have been observed to accelerate particles with fields over 100 GV/m. Such fields are three orders of magnitude higher than the breakdown limit of a state-of-the-art linear accelerator. The excitation of the plasma waves that support these fields can be accomplished by use of intense lasers (plasma beatwave acceleration, laser wakefield acceleration) or by injection of an intense electron beam into a plasma (plasma wakefield acceleration, or PWFA). Both varieties of acceleration are studied by the PBPL and its collaborators. Intense electron beams may additionally be focused under the influence of plasma-induced fields with strengths unequaled by other methods. A particularly promising regime of the PWFA was proposed in the PBPL, the "blow-out regime". This regime, where the electron beam is denser than the plasma, works by the production of a plasma-electron rarefied region. This region, while created by nonlinear plasma motion, contains quite linear focusing and acceleration fields. The nonlinearity of the plasma wave in the blow-out regime can be exploited to create "wave-breaking", which may give rise to trapping of plasma electrons, and a new form of super-high-brightness electron source.
Gamma-Ray spectroscopy at FACET-II
Plasma wakefield accelerators (PWFAs) provide unique opportunities for the generation of high-quality, short-pulse electron beams which are an ideal basis for high-quality radiation generation. For an underdense plasma, where the plasma density is less than the beam density, plasma electrons are blown out to approximately the plasma skin depth. Within the bubble formed by this blowout, the uniformly distributed plasma ions generate strong, linear, transverse focusing fields. The betatron motion of beam electrons in these fields generates characteristic X-ray radiation. Betatron radiation from plasma accelerators can be used to create novel compact X-ray light sources as well as for single-shot nondestructive diagnostics of beam-plasma interactions.
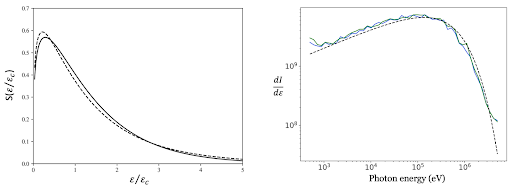
The transverse acceleration of very energetic charged particles provokes electromagnetic radiative processes, generally described as synchrotron radiation. PWFAs have already demonstrated acceleration gradients orders of magnitude higher than the limits of conventional radio-frequency acceleration and are envisioned as the core technology for next-generation, compact high-energy particle accelerators. FACET at SLAC National Accelerator Laboratory, the predecessor facility to FACET-II, achieved several key milestones toward realizing practical PWFAs including high-efficiency acceleration, high total energy gain, positron acceleration, and the demonstration of a plasma photocathode, also known as Trojan horse. The FACET-II facility aims to build on these accomplishments by demonstrating high beam quality of accelerated and plasma-injected beams and testing new concepts for acceleration, manipulation, and radiation generation. The characterization of plasma wakefield acceleration experiments using emitted photons from betatron radiation requires numerical models in support of instrumentation of single-shot, double-differential angular-energy spectra. We developed a numerical model for betatron radiation from plasma accelerated beams, that are based on the integration of Li ́enard–Wiechert (LW) potentials for computed particle trajectories.
Machine learning-based reconstruction
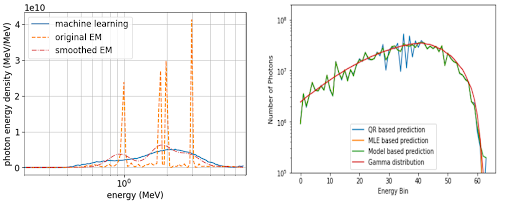
The photon flux resulting from a high-energy electron beam’s interaction with a target should yield, through its spectral and angular characteristics, information about the electron beam’s underlying dynamics at the interaction point. The data from these two cases provide a large range of photon energies; this variation of characteristics serves to increase confidence in each of the analysis methods presented. In both cases, we compared the performance of maximum likelihood estimation (MLE), a statistical technique used to determine unknown parameters from the distribution of observed data, neural networks, which detect patterns between data sets through repeated training, and a hybrid approach combining the two. Furthermore, in the case of higher energy photons, above 30 MeV, where electron-positron production is used as the basis of the spectrometer, we also examined the efficacy of QR decomposition, a matrix decomposition method. The betatron radiation case demonstrates the effectiveness of a hybrid ML-MLE approach, while the high-field electrodynamics interaction case illustrates the effectiveness of the machine learning model in the presence of noise. As such, the ML-MLE hybrid approach proves to be the most generalizable of the methods.
Examining the results above, all of these approaches provide similar performance levels, with the QR decomposition method requiring the least amount of calculational time to implement given its non-iterative algorithm in conjunction with least squares optimization. In addition, the ML and ML-MLE hybrid approaches present highly similar performance levels (with the hybrid approach providing somewhat smoother solutions). All three methods are presented in the quantum electrodynamics test case together.
Mithra Commissioning Plans
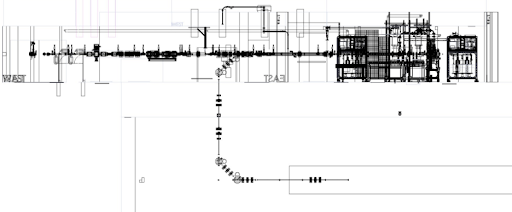
The UCLA LAPD (MITHRA) laboratory houses a hybrid photoinjector projected to be capable of producing 250pC beams with hundreds of amps of peak current and ~1 μm emittance. This source of dense electron bunches, when transported into the adjacent LAPD plasma column, can excite waves in plasma which can be resolved at high precision as they propagate over the length of the column (100ns). This long-time-scale characterization of plasma wakes is novel, and since the plasma is strongly magnetized, data would be valuable for research into space plasmas/ signal propagation in the ionosphere
Transversely asymmetric beam plasma interaction
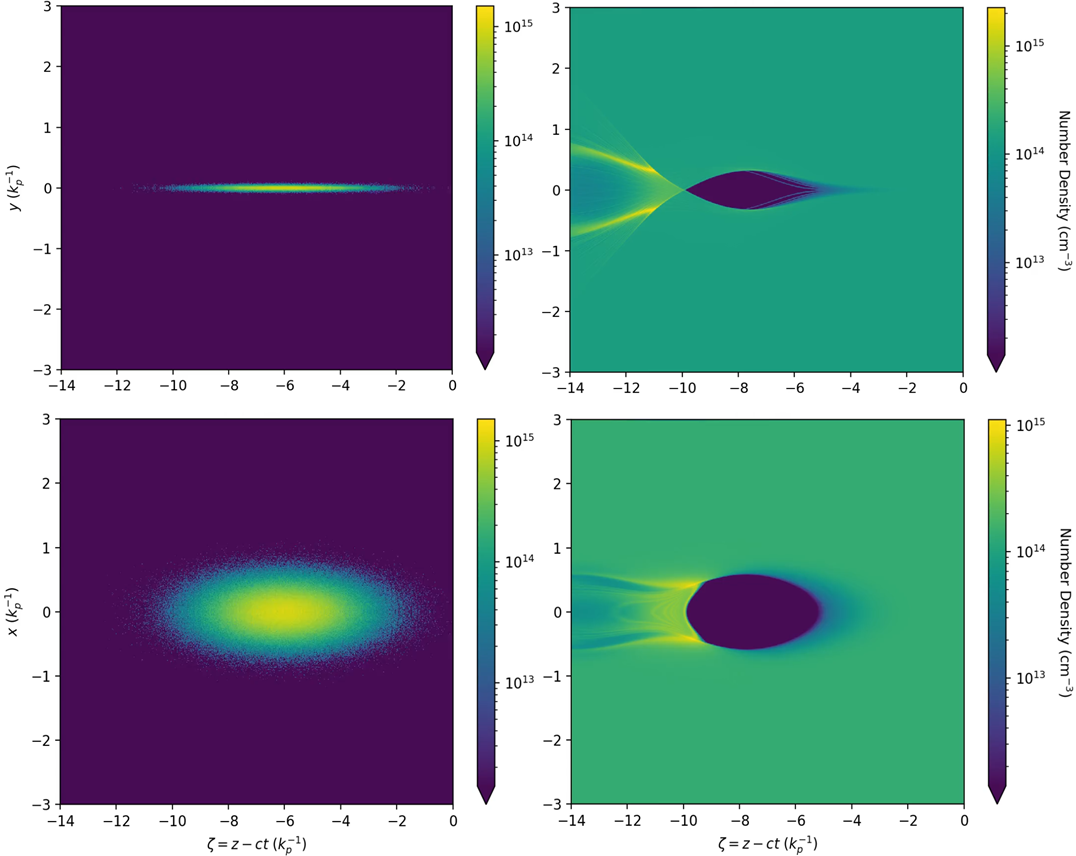
Particle beams with highly asymmetric emittance ratios, or flat beams, are employed at accelerator facilities such as the Argonne Wakefield Accelerator facility and foreseen at FACET-II at SLAC. Flat beams have been used to drive wakefields in dielectric structures and are an ideal candidate for high-gradient wakefields in plasmas. The high aspect ratio produces a blowout region that is elliptical in cross section and this asymmetry in the ion column structure creates asymmetric focusing in the two transverse planes. The ellipticity of the plasma blowout decreases as the normalized peak current increases, and gradually approaches an axisymmetric column. We are working on developing an appropriate matching condition for the beam envelope inside the elliptical blowout column. Simulations are performed to investigate the ellipticity of the resultant wakefield based on the initial drive beam parameters, and are compared to analytical calculations. Experiments will be conducted at AWA to create this asymmetric beam plasma interaction in the near future.
Ion motion
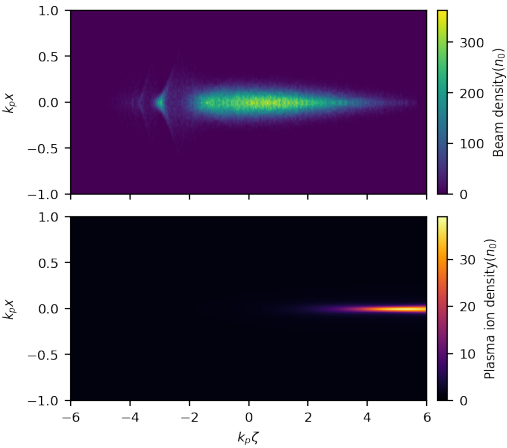
A future plasma based linear collider has the potential to reach unprecedented energies and transform our understanding of high energy physics. The extremely dense beams in such a device would cause the plasma ions to fall toward the axis. For more mild ion motion, this introduces a nonlinear perturbation to the focusing fields inside of the bubble. However, for extreme ion motion, the ion distribution collapses to a quasi-equilibrium characterized by a thin filament of extreme density on the axis which generates strong, nonlinear focusing fields. These fields can provoke unacceptable emittance growth that can be reduced through careful beam matching. The FACET-II facility offers the unique opportunity to study low emittance, GeV beams and their interactions with these high density plasmas in plasma wakefield acceleration (PWFA) scenarios. One of the experiments relevant to this is the ion collapse experiment E-314, which aims to study how ion motion in a PWFA can produce dual-focused equilibrium. As nonlinear focusing effects due to nonuniform ion distributions have not been extensively studied; we explore the difficulties of inducing ion motion in an adiabatic plasma and examine the effect an ion column has on beam focusing.
Resonant excitation
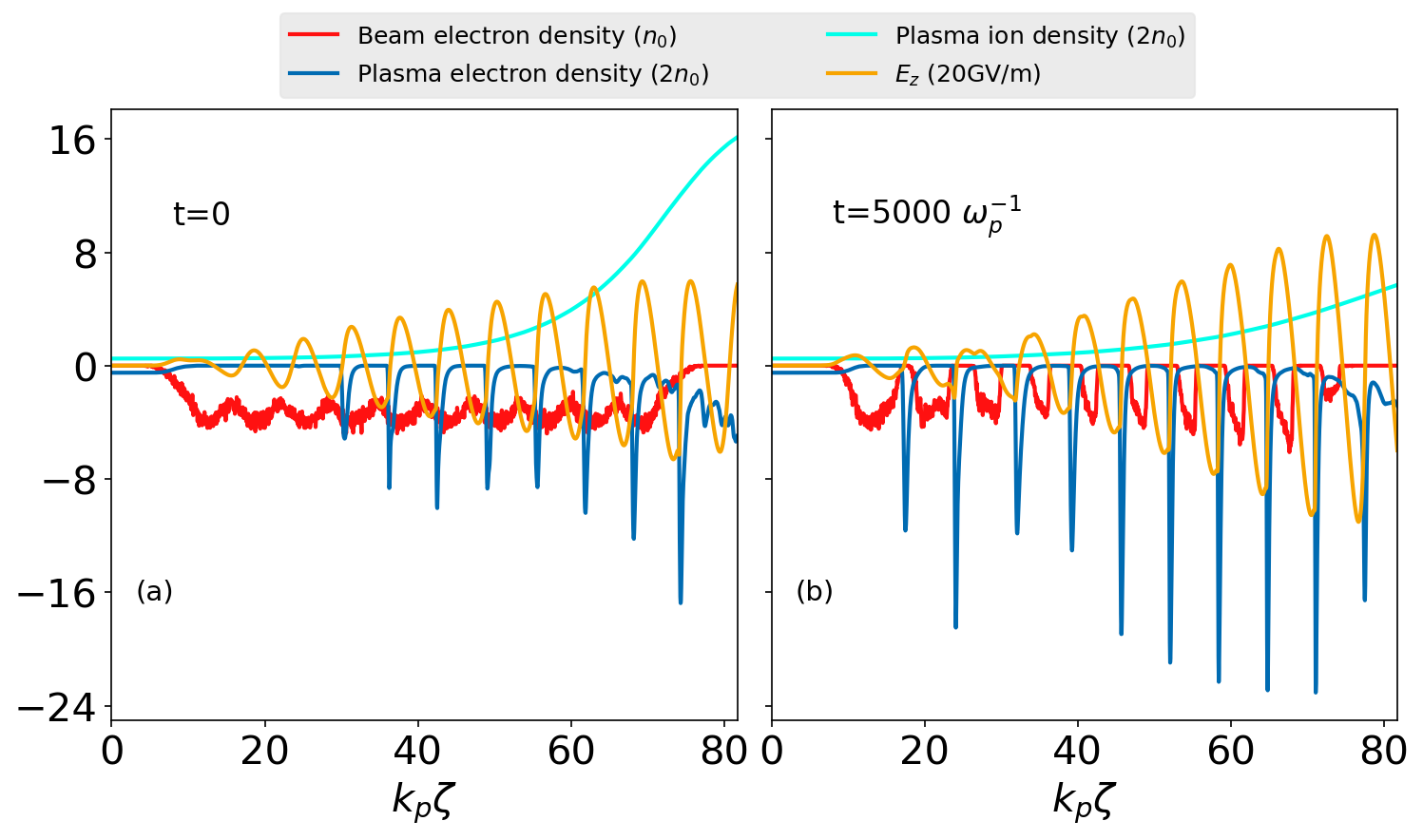
The use of a periodic electron beam bunch train to resonantly excite plasma wakefields in the quasi nonlinear (QNL) regime has distinct advantages over employing a single, higher charge bunch. Resonant QNL excitation can produce plasma electron blowout using a small charge per pulse if the beam emittance beams are very low. The local density perturbation in such a case is extremely nonlinear, achieving total rarefaction, yet the resonant response of the plasma electrons at the plasma frequency is preserved. The needed electron beam pulse train with interbunch spacing equal to the plasma period can be produced via inverse free-electron laser bunching. As such, in achieving resonance with a laser wavelength of a few microns, a high plasma density is employed, with the attendant possibility of obtaining extremely large wakefield amplitudes, near 1 TV/m for FACET-II parameters. We explore the plasma response and instabilities encountered when this bunched beam structure is used to drive a dense plasma resonantly.
Space Plasma
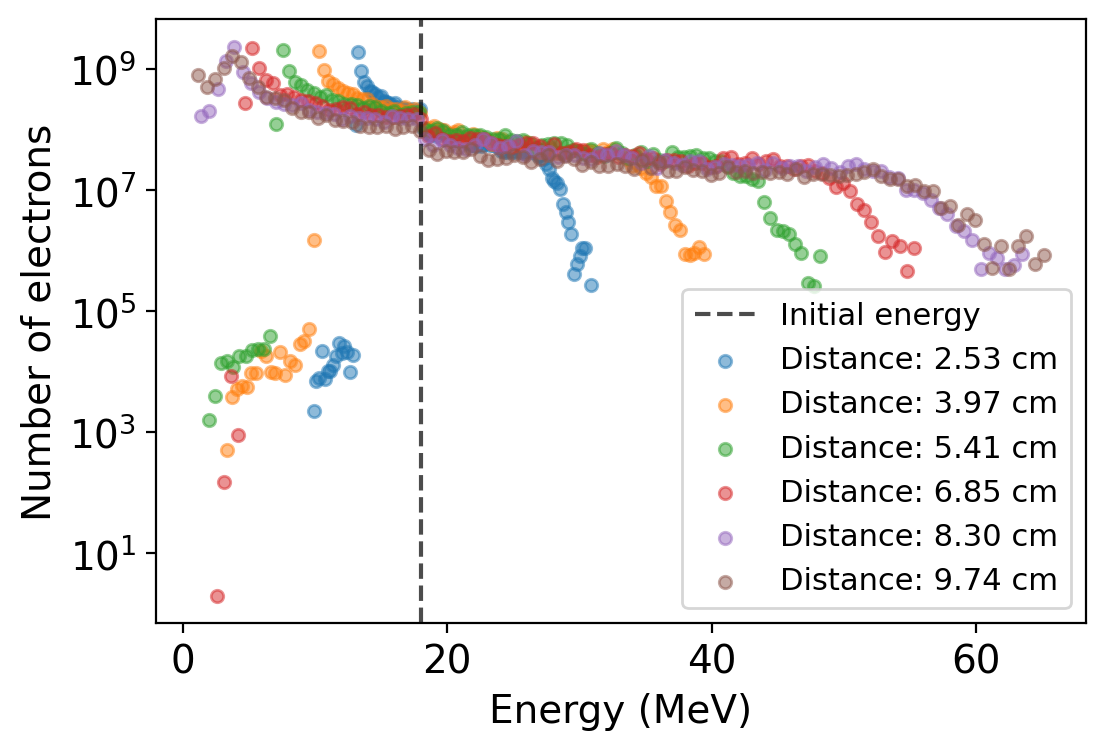
Plasma wakefield acceleration using the electron linear accelerator test facility, SAMURAI, can be used to study the Jovian electron spectrum due to the high energy spread of the beam after the plasma interaction. The SAMURAI RF facility which is currently being constructed and commissioned at UCLA, will be capable of producing beams with 100s pC of charge with bunch lengths in the 100s of fs range with low transverse emittances in the 3-80 MeV range. This study focuses on the generation of broadband electron energy spectra using a beam-driven plasma wakefield accelerator (PWFA), that replicates specific characteristics of the Jovian electron spectrum. This can be beneficial for space research and development as it can serve as a proxy for electron radiation exposure which can cause damage to high-end equipment and electronics used in satellites and flyby operations.