Beam Production
Electrons used in linear accelerators are generated via materials known as cathodes. At PBPL we study emission physics from a theoretical perspective in order to study cathode behavior in order to improve beam brightness, current, and other figures of merit.
Ab-initio Simulations of Electron Rescattering and High Harmonic Generation at Nanostructured Cathodes
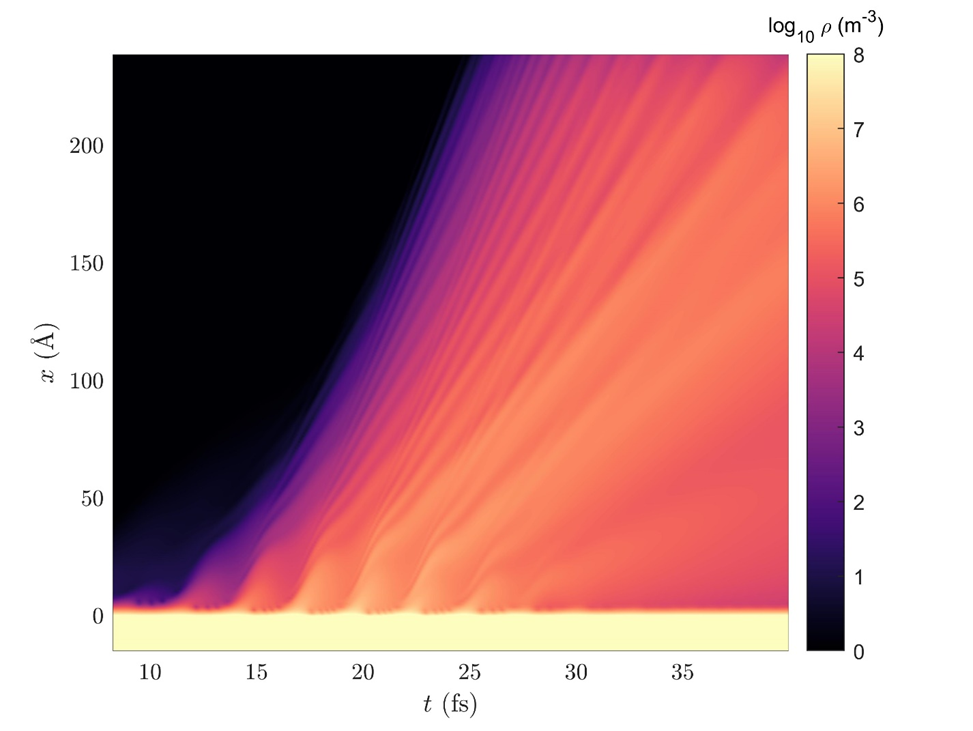
Experiments involving nanostructured cathodes are achieving peak surface fields upwards of 80 V/nm as determined by the electron emission energies.
There is thus a need to study and understand the dynamics of electrons in these extreme conditions which lie on the boundary of classical and quantum physics.
This project aims to do just that by performing time-dependent density-functional theory simulations of the interaction site with the ultimate goal of predicting yield and various beam properties such as brightness.
To date we have a 1-D simulation which includes a projected Hartree potential to model the collective image potential and space-charge trapping, the processes which limits current in the extreme high field case.
We hope to move to a full 3-D TD-DFT calculation to model the process as accurately as reasonably possible.
Incorporation of Simple Models for Strong Field Emission
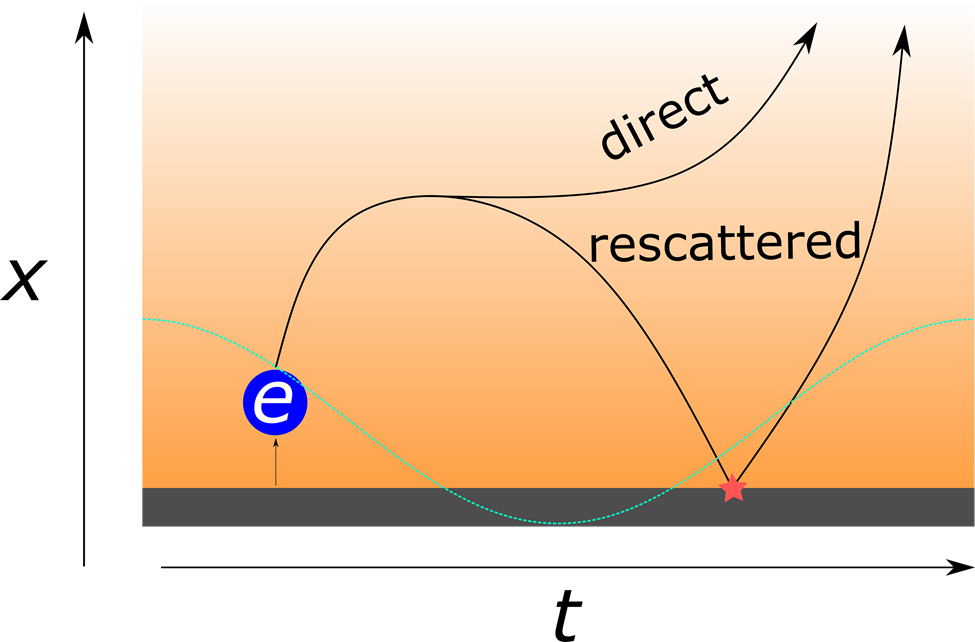
Strong laser field emission from metals is a steadily growing area of interest in the field of accelerator physics. Studying these systems accurately may involve expansive computational workloads which can obfuscate the fundamental processes underlying field emission. There has been much recent work in analytically solving the TDSE for simple systems relevant for strong field emission. There is also a significant amount of semi-classical work that may be done. This set of projects focuses on evaluating these systems in a manner which produces results relevant for accelerator physics, including yield scaling and various electron beam properties.
Ab-Initio Construction of a Material Normal Energy Distribution for Cathode Surfaces
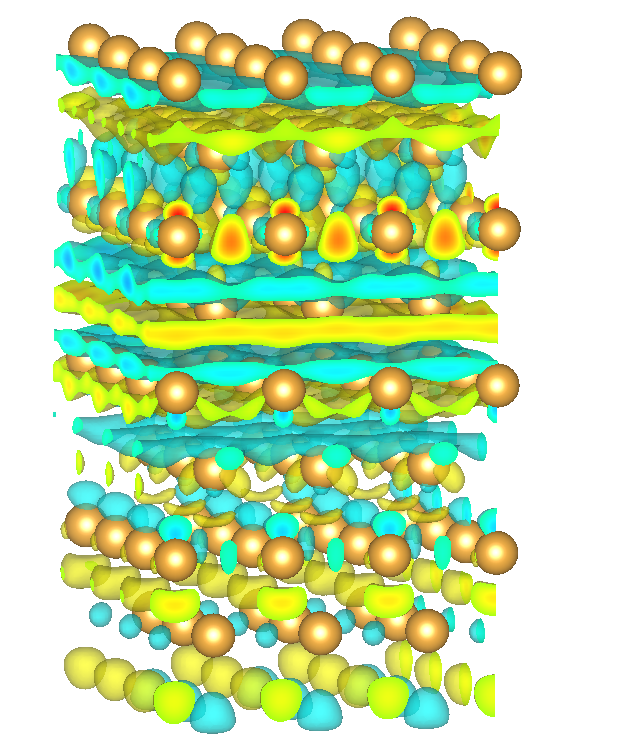
Under the influence of a strong electric field electrons may tunnel through the surface of a material. The source current and tunneling ability is ultimately dependent on the electronic dynamics within the material. Most commonly the electron distribution is summarized with the density of states (DOS) with respect to the total state energy. While relevant to perturbative photoemission, the DOS does not include sufficient information about the directionality of field emission. Therefore, we propose the ab-initio concept of a material normal energy distribution (MNED), which details the distribution of electrons with respect to an effective motive energy. We use density-functional theory (DFT) to estimate the MNED using various approaches ranging from bulk and surface analyses. We will compare the tunneling current obtained from the MNED to previous theoretical and experimental results using the Fowler-Nordheim framework for static fields and our in-house simulations for pulsed laser fields.
Nanostructured Cathodes
High brightness cathodes are increasingly a focus for accelerator applications ranging from free electron lasers to ultrafast electron diffraction. There is further an increasing interest in fabrication and control of cathode surface to better control the emission characteristics and improve beam brightness. One method which we can consider is based on well-known silicon nanofabrication techniques which we use to create patterned cathode surfaces. The sharp edges produced lead to field emission increases and high brightness emission. We have demonstrated that a beam can be successfully extracted with a low emittance and we have reconstructed a portion of the energy spectrum. Due to the simplicity of extended geometries in nanofabrication our beam uniquely possesses a high aspect ratio in its transverse cross section. We can begin to consider modifications for emittance exchange beamlines and having shown the patterning principle is sound we can consider additional patterns such as hollow beams. Future work will continue to characterize the produced beam and the addition of fabrication steps to remove one of the blades in the double blade geometry in order to more accurately characterize the emission.
Cryogenic Photoemission
There are many potentially advantageous properties of photocathodes at cryogenic temperatures. We will here define cryogenic temperatures as those below 100K. Using the three step model shown in the figure to the right, Dowell and Schmerge showed that well-above photoemission threshold, where the photon energy is much greater than the work function, the temperature of the photoemitted electrons scales as the difference between the photon energy and the cathode work function which we call the excess energy. The quantum efficiency or QE which is the ratio of emitted electrons to absorbed photons scales as the square of the excess energy.
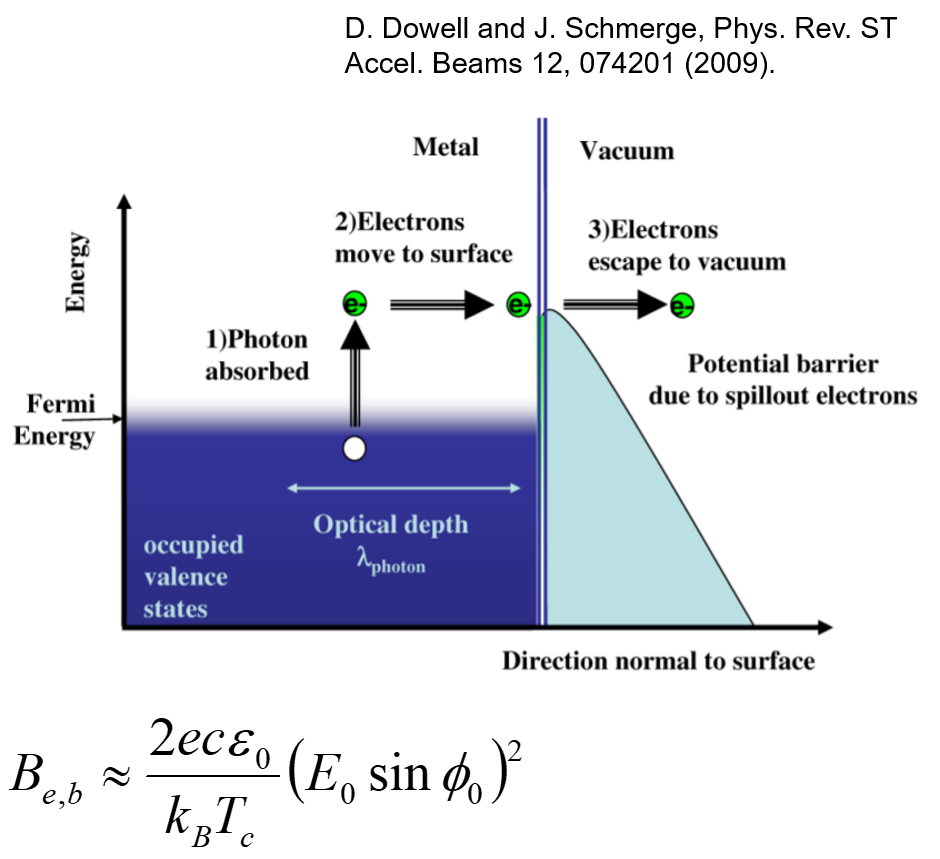
For copper photocathodes the emission temperature corresponds to around 100 meV up to the 1eV, depending on the wavelength of illuminating photons. We can also note the beam brightness should scale as the inverse of the photoemission temperature which is especially important considering our primary use case of the UCXFEL and the importance of the 6 dimensional beam brightness in the FEL performance. The real physics of more advanced cathodes is less well understood but we intend to study emission physics in order to realize these advantages. The primary device for these studies is the CYBORG beamline at the MOTHRA lab.