Beam-Radiation Interaction
Charged particles radiate whenever accelerated by a variety of mechanisms, among them synchrotron (and undulator) radiation, transition radiation, Cerenkov radiation and new effects in plasma, such as betatron radiation. PBPL research is heavily concentrated on the development and use of this challenging systems as they grow from physical concepts to transformational instruments in application. At the cutting edge of particle beam physics, the beams are very intense, and have short pulses, ranging down to the femtosecond level. Whatever the radiation mechanism, in our laboratory experiments the phenomena we are most concerned with are linked by the characteristic that the beam particles radiate together coherently, and the radiation can be enhanced by 8 or 9 orders of magnitude. In certain scenarios, coherent radiation is advantageous for the purposeful production of intense, monochromatic radiation -- free electron lasers are a good example. Conversely, the inverse free-electron laser (IFEL) is a mechanism by which we can use intense laser fields to accelerate charged particles at high gradient. Even the collision of intense electron beams with intense laser beams in vacuum may produce highly Doppler shifted radiation – photons up to the MeV energy level – via the inverse Compton scattering mechanism. This scheme, along with the FEL, forms the basis of new generations of fs-to-ps x-ray light sources. At the PBPL we are uniquely engaged in exploring the marriage of advanced, high field accelerators and light sources with game-changing characteristics.
UCXFEL
In the field of beam physics, two frontier topics have taken center stage due to their potential to enable new approaches to discovery in a wide swath of science. These areas are: advanced, high gradient acceleration techniques, and x-ray free electron lasers (XFELs). Further, there is intense interest in the marriage of these two fields, with the goal of producing a very compact XFEL. In this context, recent advances in high gradient radio-frequency cryogenic copper structure research have opened the door to the use of surface electric fields between 250 and 500 MV m−1. Such an approach is foreseen to enable a new generation of photoinjectors with six-dimensional beam brightness beyond the current state-of-the-art by well over an order of magnitude. This advance is an essential ingredient enabling an ultra-compact XFEL (UC-XFEL). In addition, one may accelerate these bright beams to GeV scale in less than 10 m. Such an injector, when combined with inverse free electron laser-based bunching techniques can produce multi-kA beams with unprecedented beam quality, quantified by 50 nm-rad normalized emittances. The emittance, we note, is the effective area in transverse phase space (x, px/mec) or (y, py/mec) occupied by the beam distribution, and it is relevant to achievable beam sizes as well as setting a limit on FEL wavelength. These beams, when injected into innovative, short-period (1–10 mm) undulators uniquely enable UC-XFELs having footprints consistent with university-scale laboratories. We describe the architecture and predicted performance of this novel light source, which promises photon production per pulse of a few percent of existing XFEL sources. We review implementation issues including collective beam effects, compact x-ray optics systems, and other relevant technical challenges. To illustrate the potential of such a light source to fundamentally change the current paradigm of XFELs with their limited access, we examine possible applications in biology, chemistry, materials, atomic physics, industry, and medicine—including the imaging of virus particles—which may profit from this new model of performing XFEL science.
FEL Development at MITHRA Lab
The ultra-compact x-ray free-electron laser (UC-XFEL), described in [J. B. Rosenzweig, et al. 2020 New J. Phys. 22 093067], combines several cutting edge beam physics techniques and technologies to realize an x-ray free electron laser at a fraction of the cost and footprint of existing XFEL installations. These elements include cryogenic, normally conducting RF structures for both the gun and linac, IFEL bunch compression, and short-period undulators. In this work, several stepping-stone, demonstrator scenarios under discussion for the UCLA SAMURAI Laboratory are detailed and simulated, employing different subsets of these elements. The cost, footprint, and technology risk for these scenarios are considered in addition to the anticipated engineering and physics experience gained.
Inverse Compton Scattering (ICS)
Inverse Compton Scattering (ICS) is an attractive pulsed radiation sources in the X to Gamma-ray spectral region at photon energy of 4γhνL, where γ is the Lorenz factor of the electron beam and hνL is the incident laser’s photon energy owing to a relativistic Doppler blue shift. Especially in BNL ATF, use of TW class relativistically intense, long wavelength, CO2 laser of peak normalized vector potential aL of approaching unity and 50-70 MeV electron beam enables us to investigate the nonlinear effects associated with the relativistic figure-8 motion due to the significance of v×B term in the Lorentz force under the strong laser fields
Compton Spectrometer at FACET-II
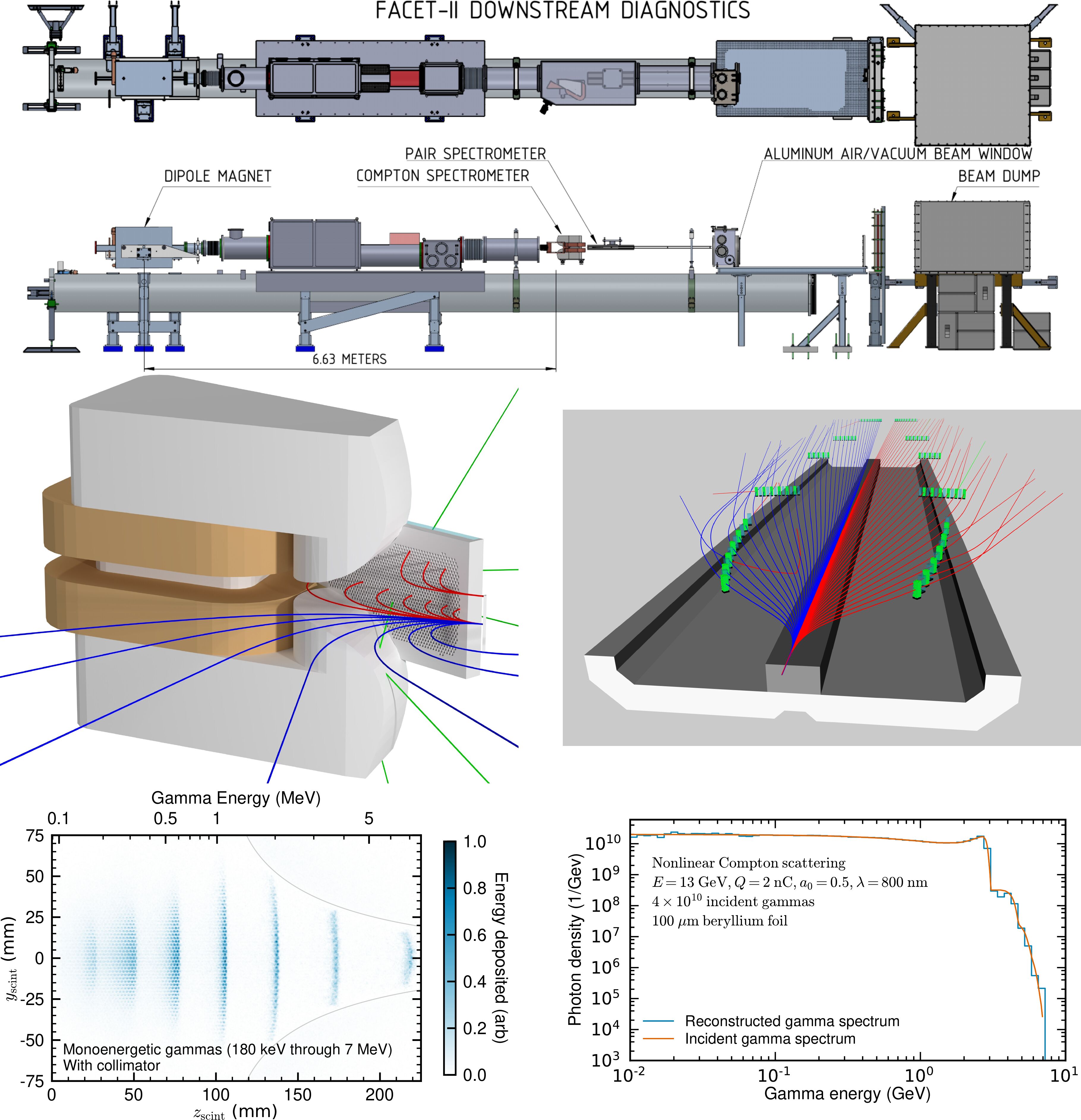
UCLA is building Compton and pair photon spectrometers to be installed at SLAC's FACET-II facility. These are single-shot spectrometers capable of collecting double-differential (energy-angle) spectra over a photon energy range of 200 keV through 10 GeV. They provide critical dynamical information of SLAC's 10 GeV electron beam interacting with a variety of targets, including: plasmas for studying plasma-wakefield accelerators, high-intensity laser pulses for studying non-perturbative QED, and solid targets for studying the relativistic streaming instabilities relevant to a variety of astrophysical processes.
X-ray laser oscillator
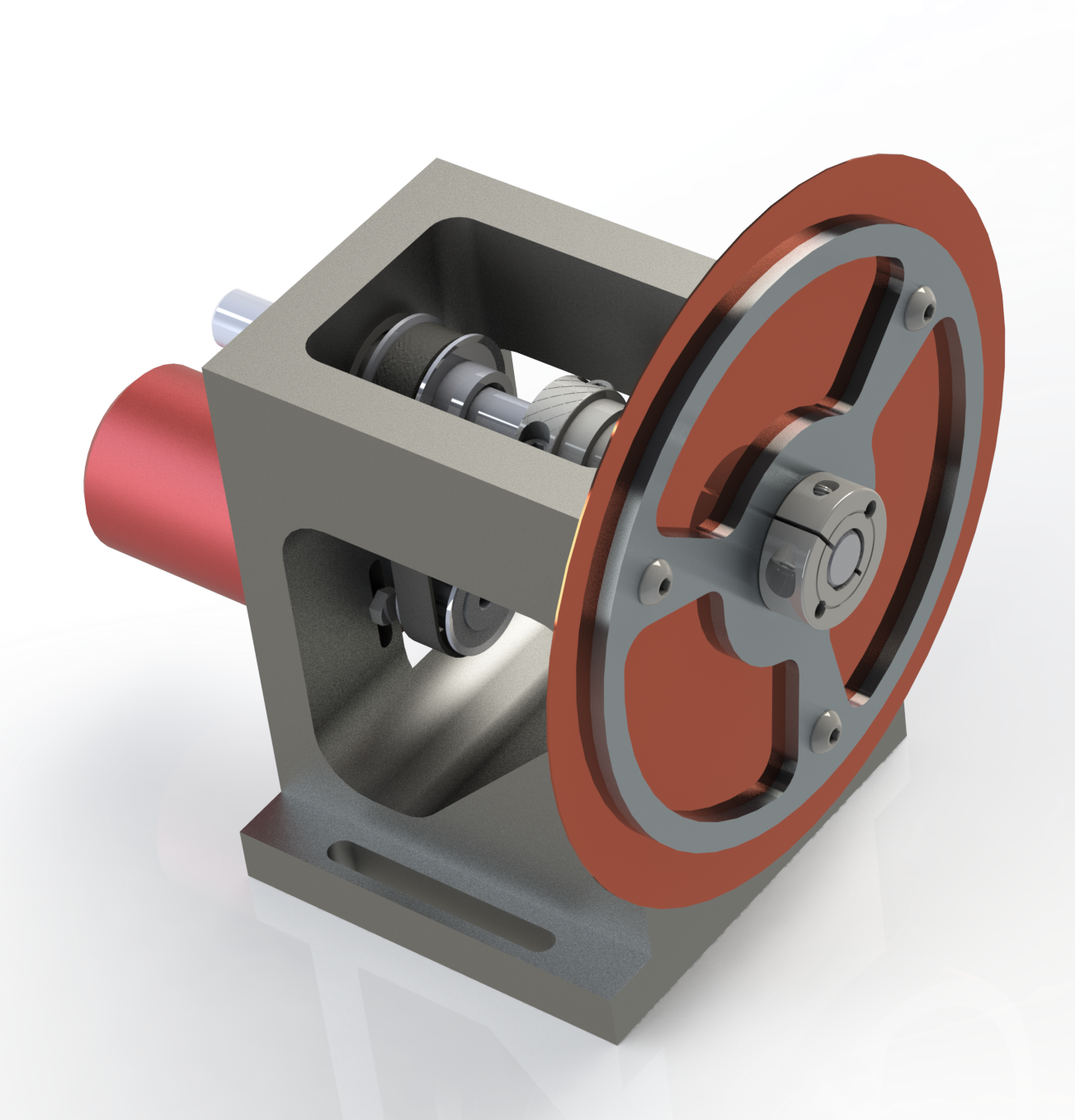
An X-ray oscillator generating fully coherent pulses at the characteristic length and time scale of atomic and molecular phenomena, angstrom wavelength, and femtosecond time duration has been a dream of laser physics from the 1960s. Our group is working on an X-ray oscillator based on population inversion of Kα electrons in a transition-metal compound, generating fully coherent, transform-limited, high-power radiation pulses, in the 3- to <1-Å wavelength region, with a high-energy resolution, about 0.05 eV, showing that the dream can now become a reality. The X-ray laser oscillator will open experimental possibilities and research areas in quantum optics, chemical dynamics, imaging, spectroscopy, and interferometric measurements. We are working on the development of the solid and liquid sample delivery systems. We are also investigating the material excitation process and crater formations to optimize the replacement of the sample material.